NISC library
NISC library
For researchers and students seeking to delve into the groundbreaking work of the NISC, our dedicated section offers a comprehensive repository of codes. This centralized hub provides easy access to a wide range of programming languages and tools utilized by NISC researchers. Whether you're interested in replicating experiments, exploring new applications, or simply learning from the expertise of NISC scientists, this collection serves as a valuable resource.
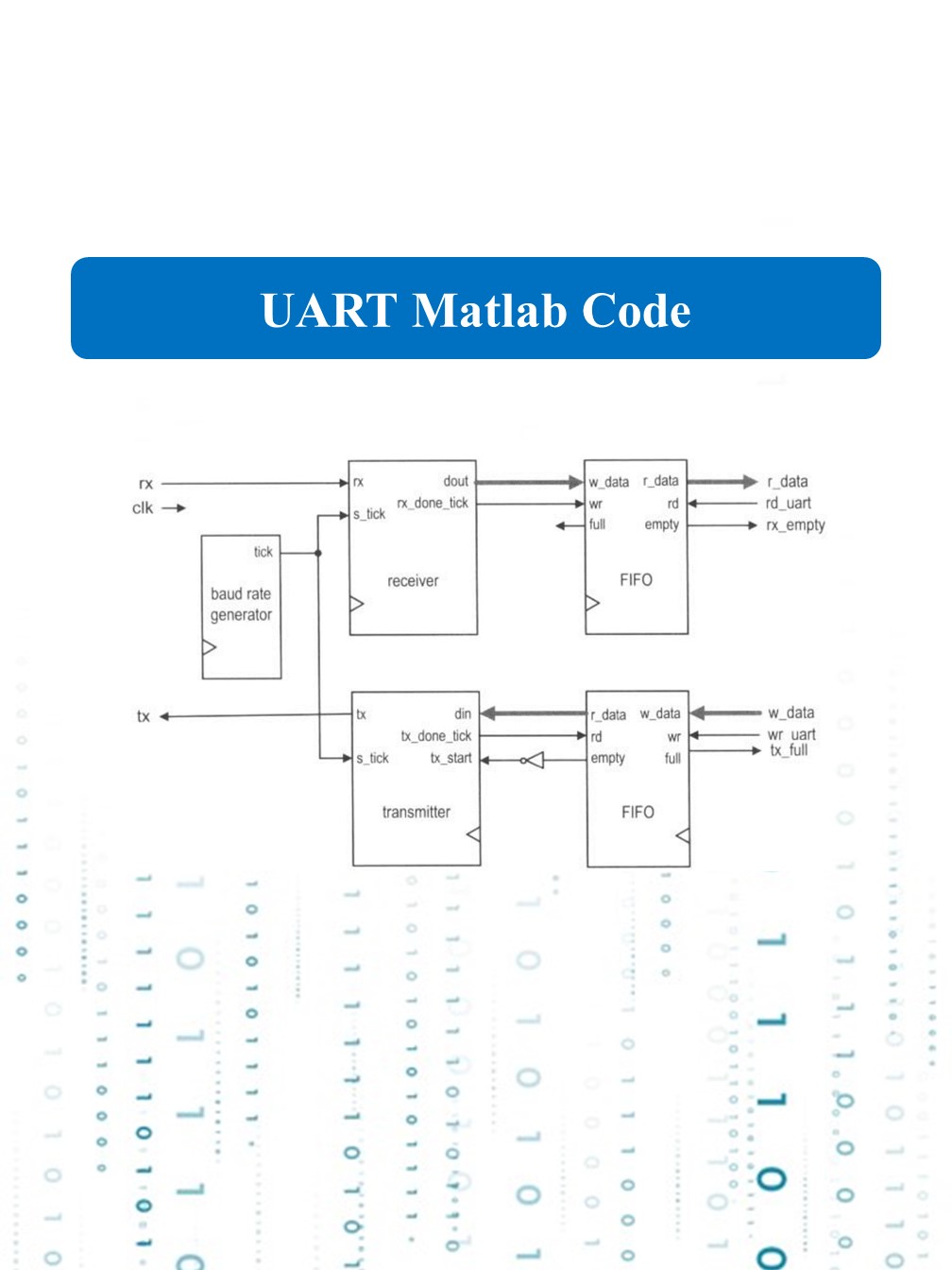
UART Matlab Code
UART Matlab Code UART (Universal Asynchronous Receiver-Transmitter) is a serial communication protocol widely used in embedded systems for short-distance, low-speed, and reliable data exchange. Unlike protocols like SPI or I2C, UART is asynchronous, meaning there is no shared clock between the sender and receiver. Here’s a detailed breakdown of how UART works and its key concepts: 1. UART Overview: UART is a hardware communication module typically built into microcontrollers that facilitates full-duplex communication (transmitting and receiving data simultaneously). Data is sent in the form of
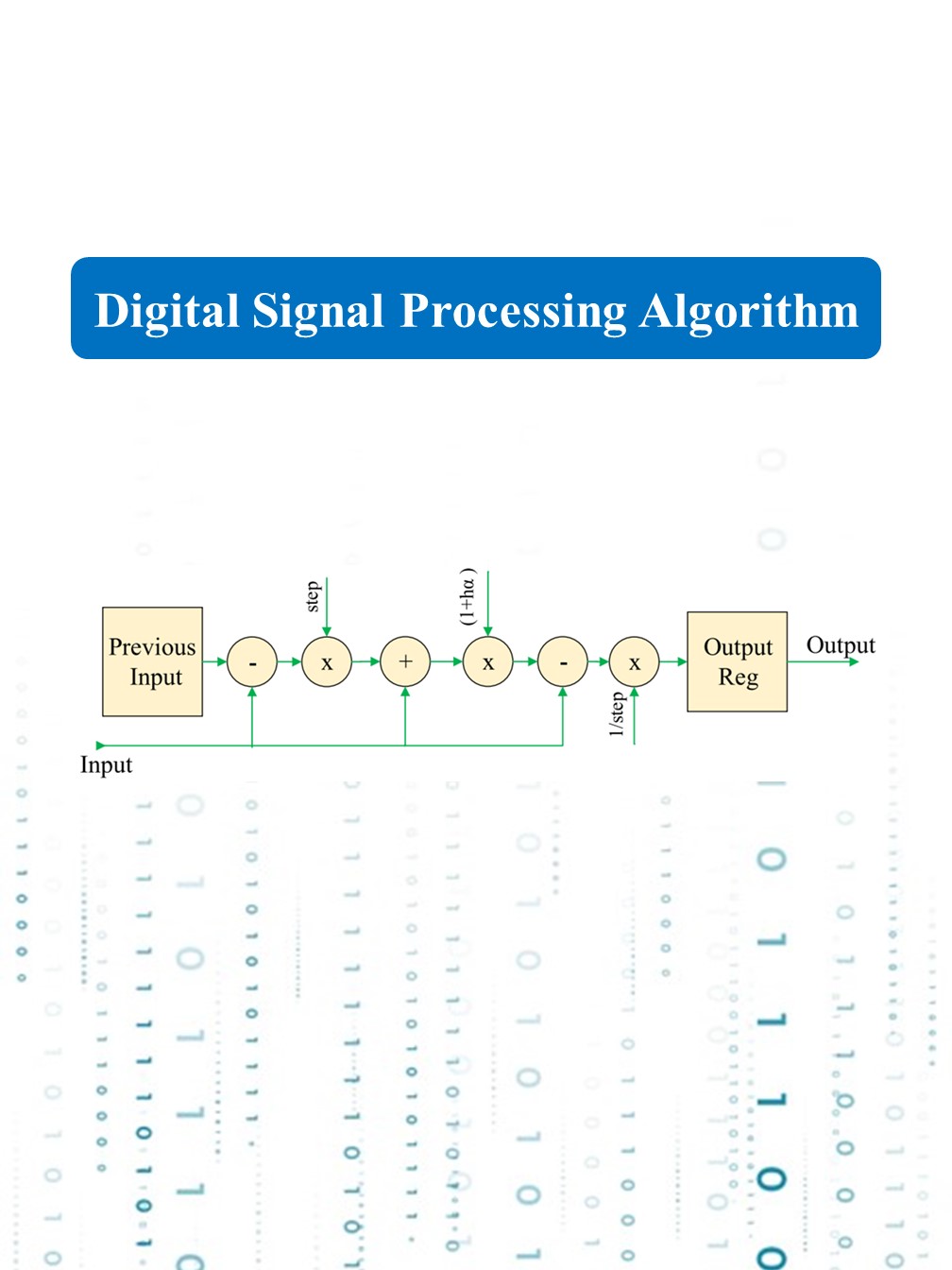
Digital Signal Processing Algorithm
Digital Signal Processing Algorithm The Deform_Frac module implements a digital signal processing algorithm that adjusts an input signal based on previous values and predefined scaling factors, effectively transforming the signal in a controlled manner. The core function of this module is to modify the signal dynamically, potentially smoothing or correcting it based on its change over time. By using fixed-point arithmetic, the module handles precise adjustments that are scaled and factored to meet specific signal processing requirements. This operation can be particularly useful in
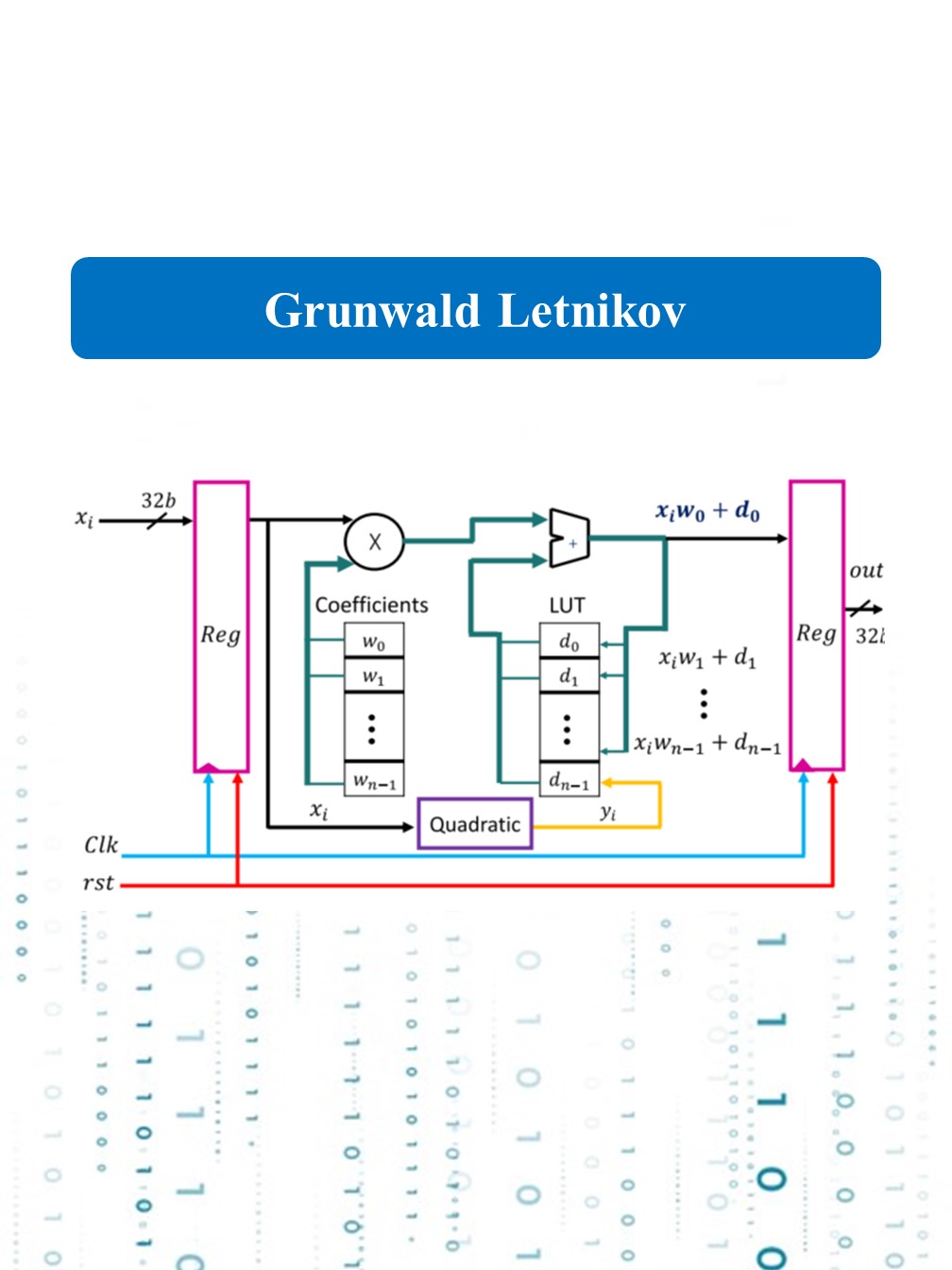
Grunwald Letnikov
Grunwald Letnikov The design and hardware implementation of the GL integrator/differentiator are illustrated in Figure. The input and output signals are 32-bit fixed-point numbers, with 8 bits for the integer part and 24 bits for the fractional part. The proposed design is divided into two main parts. The first part is the fixed window, which includes the multiplication of coefficients W0 through W(n-1) with the input signal, as presented in Fig. 7. The second part is the quadratic approximation, which computes the output based on a quadratic equation. The first part of the design uses two